Coral
Corals Temporal range:
| |
---|---|
![]() | |
A coral outcrop on the Great Barrier Reef, Australia | |
Scientific classification ![]() | |
Domain: | Eukaryota |
Kingdom: | Animalia |
Phylum: | Cnidaria |
Subphylum: | Anthozoa Ehrenberg, 1834 |
Subdivisions | |
Corals are colonial marine invertebrates within the subphylum Anthozoa of the phylum Cnidaria. They typically form compact colonies of many identical individual polyps. Coral species include the important reef builders that inhabit tropical oceans and secrete calcium carbonate to form a hard skeleton.
A coral "group" is a colony of very many genetically identical polyps. Each polyp is a sac-like animal typically only a few millimeters in diameter and a few centimeters in height. A set of tentacles surround a central mouth opening. Each polyp excretes an exoskeleton near the base. Over many generations, the colony thus creates a skeleton characteristic of the species which can measure up to several meters in size. Individual colonies grow by asexual reproduction of polyps. Corals also breed sexually by spawning: polyps of the same species release gametes simultaneously overnight, often around a full moon. Fertilized eggs form planulae, a mobile early form of the coral polyp which, when mature, settles to form a new colony.
Although some corals are able to catch plankton and small fish using stinging cells on their tentacles, most corals obtain the majority of their energy and nutrients from photosynthetic unicellular dinoflagellates of the genus Symbiodinium that live within their tissues. These are commonly known as zooxanthellae and give the coral color. Such corals require sunlight and grow in clear, shallow water, typically at depths less than 60 metres (200 feet; 33 fathoms), but corals in the genus Leptoseris have been found as deep as 172 metres (564 feet; 94 fathoms).[1] Corals are major contributors to the physical structure of the coral reefs that develop in tropical and subtropical waters, such as the Great Barrier Reef off the coast of Australia. These corals are increasingly at risk of bleaching events where polyps expel the zooxanthellae in response to stress such as high water temperature or toxins.
Other corals do not rely on zooxanthellae and can live globally in much deeper water, such as the cold-water genus Lophelia which can survive as deep as 3,300 metres (10,800 feet; 1,800 fathoms).[2] Some have been found as far north as the Darwin Mounds, northwest of Cape Wrath, Scotland, and others off the coast of Washington state and the Aleutian Islands.
Taxonomy
[edit]The classification of corals has been discussed for millennia, owing to having similarities to both plants and animals. Aristotle's pupil Theophrastus described the red coral, korallion, in his book on stones, implying it was a mineral, but he described it as a deep-sea plant in his Enquiries on Plants, where he also mentions large stony plants that reveal bright flowers when under water in the Gulf of Heroes.[3] Pliny the Elder stated boldly that several sea creatures including sea nettles and sponges "are neither animals nor plants, but are possessed of a third nature (tertia natura)".[4] Petrus Gyllius copied Pliny, introducing the term zoophyta for this third group in his 1535 book On the French and Latin Names of the Fishes of the Marseilles Region; it is popularly but wrongly supposed that Aristotle created the term.[4] Gyllius further noted, following Aristotle, how hard it was to define what was a plant and what was an animal.[4] The Babylonian Talmud refers to coral among a list of types of trees, and the 11th-century French commentator Rashi describes it as "a type of tree (מין עץ) that grows underwater that goes by the (French) name 'coral'."[5]
The Persian polymath Al-Biruni (d.1048) classified sponges and corals as animals, arguing that they respond to touch.[6] Nevertheless, people believed corals to be plants until the eighteenth century when William Herschel used a microscope to establish that coral had the characteristic thin cell membranes of an animal.[7]
Presently, corals are classified as species of animals within the sub-classes Hexacorallia and Octocorallia of the class Anthozoa in the phylum Cnidaria.[8] Hexacorallia includes the stony corals and these groups have polyps that generally have a 6-fold symmetry. Octocorallia includes blue coral and soft corals and species of Octocorallia have polyps with an eightfold symmetry, each polyp having eight tentacles and eight mesenteries. The group of corals is paraphyletic because the sea anemones are also in the sub-class Hexacorallia.
get a life idea stealer
Systematics
[edit]![]() | This section's use of external links may not follow Wikipedia's policies or guidelines. (August 2023) |
The delineation of coral species is challenging as hypotheses based on morphological traits contradict hypotheses formed via molecular tree-based processes.[9] As of 2020, there are 2175 identified separate coral species, 237 of which are currently endangered,[10] making distinguishing corals to be the utmost of importance in efforts to curb extinction.[9] Adaptation and delineation continues to occur in species of coral[11] in order to combat the dangers posed by the climate crisis. Corals are colonial modular organisms formed by asexually produced and genetically identical modules called polyps. Polyps are connected by living tissue to produce the full organism.[12] The living tissue allows for inter module communication (interaction between each polyp),[12] which appears in colony morphologies produced by corals, and is one of the main identifying characteristics for a species of coral.[12]
There are two main classifications for corals: hard coral (scleractinian and stony coral)[13] which form reefs by a calcium carbonate base, with polyps that bear six stiff tentacles,[14] and soft coral (Alcyonacea and ahermatypic coral)[13] which are pliable and formed by a colony of polyps with eight feather-like tentacles.[14] These two classifications arose from differentiation in gene expressions in their branch tips[12] and bases that arose through developmental signaling pathways such as Hox, Hedgehog, Wnt, BMP etc.
Scientists typically select Acropora as research models since they are the most diverse genus of hard coral, having over 120 species.[12] Most species within this genus have polyps which are dimorphic:[15] axial polyps grow rapidly and have lighter coloration, while radial polyps are small and are darker in coloration.[12][16] In the Acropora genus, gamete synthesis and photosynthesis occur at the basal[17] polyps, growth occurs mainly at the radial polyps. Growth at the site of the radial polyps encompasses two processes: asexual reproduction via mitotic cell proliferation,[12] and skeleton deposition of the calcium carbonate via extra cellular matrix (EMC) proteins acting as differentially expressed (DE) signaling genes[12] between both branch tips and bases. These processes lead to colony differentiation, which is the most accurate distinguisher between coral species.[9] In the Acropora genus, colony differentiation through up-regulation and down-regulation of DEs.[12]
Systematic studies of soft coral species have faced challenges due to a lack of taxonomic knowledge.[9] Researchers have not found enough variability within the genus to confidently delineate similar species, due to a low rate in mutation of mitochondrial DNA.[18]
Environmental factors, such as the rise of temperatures and acid levels in our oceans account for some speciation of corals in the form of species lost.[12] Various coral species have heat shock proteins (HSP) that are also in the category of DE across species.[12] These HSPs help corals combat the increased temperatures they are facing which lead to protein denaturing, growth loss, and eventually coral death.[12] Approximately 33% of coral species are on the International Union for Conservation of Nature's endangered species list and at risk of species loss.[19] Ocean acidification (falling pH levels in the oceans) is threatening the continued species growth and differentiation of corals.[12] Mutation rates of Vibrio shilonii, the reef pathogen responsible for coral bleaching, heavily outweigh the typical reproduction rates of coral colonies when pH levels fall.[20] Thus, corals are unable to mutate their HSPs and other climate change preventative genes to combat the increase in temperature and decrease in pH at a competitive rate to these pathogens responsible for coral bleaching,[20] resulting in species loss.
Anatomy
[edit]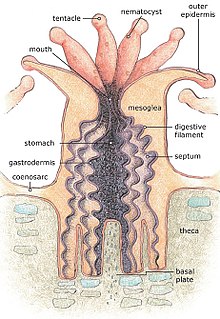
For most of their life corals are sessile animals of colonies of genetically identical polyps. Each polyp varies from millimeters to centimeters in diameter, and colonies can be formed from many millions of individual polyps. Stony coral, also known as hard coral, polyps produce a skeleton composed of calcium carbonate to strengthen and protect the organism. This is deposited by the polyps and by the coenosarc, the living tissue that connects them. The polyps sit in cup-shaped depressions in the skeleton known as corallites. Colonies of stony coral are markedly variable in appearance; a single species may adopt an encrusting, plate-like, bushy, columnar or massive solid structure, the various forms often being linked to different types of habitat, with variations in light level and water movement being significant.[21]
The body of the polyp may be roughly compared in a structure to a sac, the wall of which is composed of two layers of cells. The outer layer is known technically as the ectoderm, the inner layer as the endoderm. Between ectoderm and endoderm is a supporting layer of gelatinous substance termed mesoglea, secreted by the cell layers of the body wall.[22] The mesoglea can contain skeletal elements derived from cells migrated from the ectoderm.
The sac-like body built up in this way is attached to a hard surface, which in hard corals are cup-shaped depressions in the skeleton known as corallites. At the center of the upper end of the sac lies the only opening called the mouth, surrounded by a circle of tentacles which resemble glove fingers. The tentacles are organs which serve both for tactile sense and for the capture of food.[22] Polyps extend their tentacles, particularly at night, often containing coiled stinging cells (cnidocytes) which pierce, poison and firmly hold living prey paralyzing or killing them. Polyp prey includes plankton such as copepods and fish larvae. Longitudinal muscular fibers formed from the cells of the ectoderm allow tentacles to contract to convey the food to the mouth. Similarly, circularly disposed muscular fibres formed from the endoderm permit tentacles to be protracted or thrust out once they are contracted.[22] In both stony and soft corals, the polyps can be retracted by contracting muscle fibres, with stony corals relying on their hard skeleton and cnidocytes for defense. Soft corals generally secrete terpenoid toxins to ward off predators.[21]
In most corals, the tentacles are retracted by day and spread out at night to catch plankton and other small organisms. Shallow-water species of both stony and soft corals can be zooxanthellate, the corals supplementing their plankton diet with the products of photosynthesis produced by these symbionts.[21] The polyps interconnect by a complex and well-developed system of gastrovascular canals, allowing significant sharing of nutrients and symbionts.[23]
The external form of the polyp varies greatly. The column may be long and slender, or may be so short in the axial direction that the body becomes disk-like. The tentacles may number many hundreds or may be very few, in rare cases only one or two. They may be simple and unbranched, or feathery in pattern. The mouth may be level with the surface of the peristome, or may be projecting and trumpet-shaped.[22]
Soft corals
[edit]Soft corals have no solid exoskeleton as such. However, their tissues are often reinforced by small supportive elements known as sclerites made of calcium carbonate. The polyps of soft corals have eight-fold symmetry, which is reflected in the Octo in Octocorallia.[24]
Soft corals vary considerably in form, and most are colonial. A few soft corals are stolonate, but the polyps of most are connected by sheets of tissue called coenosarc, and in some species these sheets are thick and the polyps deeply embedded in them. Some soft corals encrust other sea objects or form lobes. Others are tree-like or whip-like and have a central axial skeleton embedded at their base in the matrix of the supporting branch.[25] These branches are composed of a fibrous protein called gorgonin or of a calcified material.
Stony corals
[edit]
The polyps of stony corals have six-fold symmetry. In stony corals, the tentacles are cylindrical and taper to a point, but in soft corals they are pinnate with side branches known as pinnules. In some tropical species, these are reduced to mere stubs and in some, they are fused to give a paddle-like appearance.[26]
Coral skeletons are biocomposites (mineral + organics) of calcium carbonate, in the form of calcite or aragonite. In scleractinian corals, "centers of calcification" and fibers are clearly distinct structures differing with respect to both morphology and chemical compositions of the crystalline units.[27][28] The organic matrices extracted from diverse species are acidic, and comprise proteins, sulphated sugars and lipids; they are species specific.[29] The soluble organic matrices of the skeletons allow to differentiate zooxanthellae and non-zooxanthellae specimens.[30]
Ecology
[edit]
Feeding
[edit]Polyps feed on a variety of small organisms, from microscopic zooplankton to small fish. The polyp's tentacles immobilize or kill prey using stinging cells called nematocysts. These cells carry venom which they rapidly release in response to contact with another organism. A dormant nematocyst discharges in response to nearby prey touching the trigger (Cnidocil). A flap (operculum) opens and its stinging apparatus fires the barb into the prey. The venom is injected through the hollow filament to immobilise the prey; the tentacles then manoeuvre the prey into the stomach. Once the prey is digested the stomach reopens allowing the elimination of waste products and the beginning of the next hunting cycle.[31]: 24
Intracellular symbionts
[edit]Many corals, as well as other cnidarian groups such as sea anemones form a symbiotic relationship with a class of dinoflagellate algae, zooxanthellae of the genus Symbiodinium, which can form as much as 30% of the tissue of a polyp.[31]: 23–24 Typically, each polyp harbors one species of alga, and coral species show a preference for Symbiodinium.[32] Young corals are not born with zooxanthellae, but acquire the algae from the surrounding environment, including the water column and local sediment.[33] The main benefit of the zooxanthellae is their ability to photosynthesize which supplies corals with the products of photosynthesis, including glucose, glycerol, also amino acids, which the corals can use for energy.[34] Zooxanthellae also benefit corals by aiding in calcification, for the coral skeleton, and waste removal.[35][36] In addition to the soft tissue, microbiomes are also found in the coral's mucus and (in stony corals) the skeleton, with the latter showing the greatest microbial richness.[37]
The zooxanthellae benefit from a safe place to live and consume the polyp's carbon dioxide, phosphate and nitrogenous waste. Stressed corals will eject their zooxanthellae, a process that is becoming increasingly common due to strain placed on coral by rising ocean temperatures. Mass ejections are known as coral bleaching because the algae contribute to coral coloration; some colors, however, are due to host coral pigments, such as green fluorescent proteins (GFPs). Ejection increases the polyp's chance of surviving short-term stress and if the stress subsides they can regain algae, possibly of a different species, at a later time. If the stressful conditions persist, the polyp eventually dies.[38] Zooxanthellae are located within the coral cytoplasm and due to the algae's photosynthetic activity the internal pH of the coral can be raised; this behavior indicates that the zooxanthellae are responsible to some extent for the metabolism of their host corals.[39] Stony Coral Tissue Loss Disease has been associated with the breakdown of host-zooxanthellae physiology.[40] Moreover, Vibrio bacterium are known to have virulence traits used for host coral tissue damage and photoinhibition of algal symbionts.[41] Therefore, both coral and their symbiotic microorganisms could have evolved to harbour traits resistant to disease and transmission.
Reproduction
[edit]Corals can be both gonochoristic (unisexual) and hermaphroditic, each of which can reproduce sexually and asexually. Reproduction also allows coral to settle in new areas. Reproduction is coordinated by chemical communication.[clarify]
Sexual
[edit]
Corals predominantly reproduce sexually. About 25% of hermatypic corals (reef-building stony corals) form single-sex (gonochoristic) colonies, while the rest are hermaphroditic.[citation needed] It is estimated more than 67% of coral are simultaneous hermaphrodites.[42]
Broadcasters
[edit]External videos | |
---|---|
![]() | |
![]() |
About 75% of all hermatypic corals "broadcast spawn"[citation needed] by releasing gametes—eggs and sperm—into the water where they meet and fertilize to spread offspring. Corals often synchronize their time of spawning. This reproductive synchrony is essential so that male and female gametes can meet. Spawning frequently takes place in the evening or at night, and can occur as infrequently as once a year, and within a window of 10–30 minutes.[43][44] Synchronous spawning is very typical on the coral reef, and often, all corals spawn on the same night even when multiple species are present.[45] Synchronous spawning may form hybrids and is perhaps involved in coral speciation.[46]

Environmental cues that influence the release of gametes into the water vary from species to species. The cues involve temperature change, lunar cycle, day length, and possibly chemical signalling.[45] Other factors that affect the rhythmicity of organisms in marine habitats include salinity, mechanical forces, and pressure or magnetic field changes.[44]
Mass coral spawning often occurs at night on days following a full moon.[43][47] A full moon is equivalent to four to six hours of continuous dim light exposure, which can cause light-dependent reactions in protein.[43][44] Corals contain light-sensitive cryptochromes, proteins whose light-absorbing flavin structures are sensitive to different types of light. This allows corals such as Dipsastraea speciosa to detect and respond to changes in sunlight and moonlight.[43][44][48]
Moonlight itself may actually suppress coral spawning. The most immediate cue to cause spawning appears to be the dark portion of the night between sunset and moonrise. Over the lunar cycle, moonrise shifts progressively later, occurring after sunset on the day of the full moon. The resulting dark period between day-light and night-light removes the suppressive effect of moonlight and enables coral to spawn.[43][47]
The spawning event can be visually dramatic, clouding the usually clear water with gametes. Once released, gametes fertilize at the water's surface and form a microscopic larva called a planula, typically pink and elliptical in shape. A typical coral colony needs to release several thousand larvae per year to overcome the odds against formation of a new colony.[49][50]
Studies suggest that light pollution desynchronizes spawning in some coral species. In areas such as the Red Sea, as many as 10 out of 50 species may be showing spawning asynchrony, compared to 30 years ago. The establishment of new corals in the area has decreased and in some cases ceased. The area was previously considered a refuge for corals because mass bleaching events due to climate change had not been observed there.[43][51] Coral restoration techniques for coral reef management are being developed to increase fertilization rates, larval development, and settlement of new corals.[52]
Brooders
[edit]Brooding species are most often ahermatypic (not reef-building) in areas of high current or wave action. Brooders release only sperm, which is negatively buoyant, sinking onto the waiting egg carriers that harbor unfertilized eggs for weeks. Synchronous spawning events sometimes occur even with these species.[45] After fertilization, the corals release planula that are ready to settle.[35]

Planulae
[edit]The time from spawning to larval settlement is usually two to three days but can occur immediately or up to two months.[53] Broadcast-spawned planula larvae develop at the water's surface before descending to seek a hard surface on the benthos to which they can attach and begin a new colony.[54] The larvae often need a biological cue to induce settlement such as specific crustose coralline algae species or microbial biofilms.[55][56] High failure rates afflict many stages of this process, and even though thousands of eggs are released by each colony, few new colonies form. During settlement, larvae are inhibited by physical barriers such as sediment,[57] as well as chemical (allelopathic) barriers.[58] The larvae metamorphose into a single polyp and eventually develops into a juvenile and then adult by asexual budding and growth.
Asexual
[edit]
Within a coral head, the genetically identical polyps reproduce asexually, either by budding (gemmation) or by dividing, whether longitudinally or transversely.
Budding involves splitting a smaller polyp from an adult.[49] As the new polyp grows, it forms its body parts. The distance between the new and adult polyps grows, and with it, the coenosarc (the common body of the colony). Budding can be intratentacular, from its oral discs, producing same-sized polyps within the ring of tentacles, or extratentacular, from its base, producing a smaller polyp.
Division forms two polyps that each become as large as the original. Longitudinal division begins when a polyp broadens and then divides its coelenteron (body), effectively splitting along its length. The mouth divides and new tentacles form. The two polyps thus created then generate their missing body parts and exoskeleton. Transversal division occurs when polyps and the exoskeleton divide transversally into two parts. This means one has the basal disc (bottom) and the other has the oral disc (top); the new polyps must separately generate the missing pieces.
Asexual reproduction offers the benefits of high reproductive rate, delaying senescence, and replacement of dead modules, as well as geographical distribution.[clarification needed][59]
Colony division
[edit]Whole colonies can reproduce asexually, forming two colonies with the same genotype. The possible mechanisms include fission, bailout and fragmentation. Fission occurs in some corals, especially among the family Fungiidae, where the colony splits into two or more colonies during early developmental stages. Bailout occurs when a single polyp abandons the colony and settles on a different substrate to create a new colony. Fragmentation involves individuals broken from the colony during storms or other disruptions. The separated individuals can start new colonies.[60]
Coral microbiomes
[edit]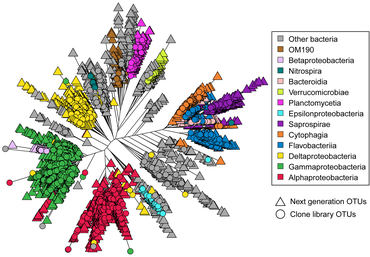
Corals are one of the more common examples of an animal host whose symbiosis with microalgae can turn to dysbiosis, and is visibly detected as bleaching. Coral microbiomes have been examined in a variety of studies, which demonstrate how oceanic environmental variations, most notably temperature, light, and inorganic nutrients, affect the abundance and performance of the microalgal symbionts, as well as calcification and physiology of the host.[62][63][64]
Studies have also suggested that resident bacteria, archaea, and fungi additionally contribute to nutrient and organic matter cycling within the coral, with viruses also possibly playing a role in structuring the composition of these members, thus providing one of the first glimpses at a multi-domain marine animal symbiosis.[65] The gammaproteobacterium Endozoicomonas is emerging as a central member of the coral's microbiome, with flexibility in its lifestyle.[66][67] Given the recent mass bleaching occurring on reefs,[68] corals will likely continue to be a useful and popular system for symbiosis and dysbiosis research.[64]
Astrangia poculata, the northern star coral, is a temperate stony coral, widely documented along the eastern coast of the United States. The coral can live with and without zooxanthellae (algal symbionts), making it an ideal model organism to study microbial community interactions associated with symbiotic state. However, the ability to develop primers and probes to more specifically target key microbial groups has been hindered by the lack of full-length 16S rRNA sequences, since sequences produced by the Illumina platform are of insufficient length (approximately 250 base pairs) for the design of primers and probes.[69] In 2019, Goldsmith et al. demonstrated Sanger sequencing was capable of reproducing the biologically relevant diversity detected by deeper next-generation sequencing, while also producing longer sequences useful to the research community for probe and primer design (see diagram on right).[70]
Holobionts
[edit]Reef-building corals are well-studied holobionts that include the coral itself together with its symbiont zooxanthellae (photosynthetic dinoflagellates), as well as its associated bacteria and viruses.[71] Co-evolutionary patterns exist for coral microbial communities and coral phylogeny.[72]

It is known that the coral's microbiome and symbiont influence host health, however, the historic influence of each member on others is not well understood. Scleractinian corals have been diversifying for longer than many other symbiotic systems, and their microbiomes are known to be partially species-specific.[74] It has been suggested that Endozoicomonas, a commonly highly abundant bacterium in corals, has exhibited codiversification with its host.[75][76] This hints at an intricate set of relationships between the members of the coral holobiont that have been developing as evolution of these members occurs.
A study published in 2018[77] revealed evidence of phylosymbiosis between corals and their tissue and skeleton microbiomes. The coral skeleton, which represents the most diverse of the three coral microbiomes, showed the strongest evidence of phylosymbiosis. Coral microbiome composition and richness were found to reflect coral phylogeny. For example, interactions between bacterial and eukaryotic coral phylogeny influence the abundance of Endozoicomonas, a highly abundant bacterium in the coral holobiont. However, host-microbial cophylogeny appears to influence only a subset of coral-associated bacteria.

AMP = antimicrobial peptides, ROS = reactive oxygen species

Reefs
[edit]
Many corals in the order Scleractinia are hermatypic, meaning that they are involved in building reefs. Most such corals obtain some of their energy from zooxanthellae in the genus Symbiodinium. These are symbiotic photosynthetic dinoflagellates which require sunlight; reef-forming corals are therefore found mainly in shallow water. They secrete calcium carbonate to form hard skeletons that become the framework of the reef. However, not all reef-building corals in shallow water contain zooxanthellae, and some deep water species, living at depths to which light cannot penetrate, form reefs but do not harbour the symbionts.[80]

There are various types of shallow-water coral reef, including fringing reefs, barrier reefs and atolls; most occur in tropical and subtropical seas. They are very slow-growing, adding perhaps one centimetre (0.4 in) in height each year. The Great Barrier Reef is thought to have been laid down about two million years ago. Over time, corals fragment and die, sand and rubble accumulates between the corals, and the shells of clams and other molluscs decay to form a gradually evolving calcium carbonate structure.[81] Coral reefs are extremely diverse marine ecosystems hosting over 4,000 species of fish, massive numbers of cnidarians, molluscs, crustaceans, and many other animals.[82]
Evolution
[edit]
At certain times in the geological past, corals were very abundant. Like modern corals, their ancestors built reefs, some of which ended as great structures in sedimentary rocks. Fossils of fellow reef-dwellers algae, sponges, and the remains of many echinoids, brachiopods, bivalves, gastropods, and trilobites appear along with coral fossils. This makes some corals useful index fossils.[84] Coral fossils are not restricted to reef remnants, and many solitary fossils are found elsewhere, such as Cyclocyathus, which occurs in England's Gault clay formation.
Early corals
[edit]Corals first appeared in the Cambrian about 535 million years ago.[85] Fossils are extremely rare until the Ordovician period, 100 million years later, when Heliolitida, rugose, and tabulate corals became widespread. Paleozoic corals often contained numerous endobiotic symbionts.[86][87]
Tabulate corals occur in limestones and calcareous shales of the Ordovician period, with a gap in the fossil record due to extinction events at the end of the Ordovician. Corals reappeared some millions of years later during the Silurian period, and tabulate corals often form low cushions or branching masses of calcite alongside rugose corals. Tabulate coral numbers began to decline during the middle of the Silurian period.[88]
Rugose or horn corals became dominant by the middle of the Silurian period, and during the Devonian, corals flourished with more than 200 genera. The rugose corals existed in solitary and colonial forms, and were also composed of calcite.[89] Both rugose and tabulate corals became extinct in the Permian–Triassic extinction event[88][90] 250 million years ago (along with 85% of marine species), and there is a gap of tens of millions of years until new forms of coral evolved in the Triassic.
-
Tabulate coral (a syringoporid); Boone limestone (Lower Carboniferous) near Hiwasse, Arkansas, scale bar is 2.0 cm
-
Solitary rugose coral (Grewingkia) in three views; Ordovician, southeastern Indiana
Modern corals
[edit]The currently ubiquitous stony corals, Scleractinia, appeared in the Middle Triassic to fill the niche vacated by the extinct rugose and tabulate orders and is not closely related to the earlier forms. Unlike the corals prevalent before the Permian extinction, which formed skeletons of a form of calcium carbonate known as calcite, modern stony corals form skeletons composed of the aragonite.[91] Their fossils are found in small numbers in rocks from the Triassic period, and become common in the Jurassic and later periods.[92] Although they are geologically younger than the tabulate and rugose corals, the aragonite of their skeletons is less readily preserved, and their fossil record is accordingly less complete.
![]() | |
Timeline of the major coral fossil record and developments from 650 m.y.a. to present.[93][94] |
Status
[edit]Threats
[edit]
Coral reefs are under stress around the world.[95] In particular, coral mining, agricultural and urban runoff, pollution (organic and inorganic), overfishing, blast fishing, disease, and the digging of canals and access into islands and bays are localized threats to coral ecosystems. Broader threats are sea temperature rise, sea level rise and pH changes from ocean acidification, all associated with greenhouse gas emissions.[96] In 1998, 16% of the world's reefs died as a result of increased water temperature.[97]
Approximately 10% of the world's coral reefs are dead.[98][99][100] About 60% of the world's reefs are at risk due to human-related activities.[101] The threat to reef health is particularly strong in Southeast Asia, where 80% of reefs are endangered.[102] Over 50% of the world's coral reefs may be destroyed by 2030; as a result, most nations protect them through environmental laws.[103]
In the Caribbean and tropical Pacific, direct contact between ~40–70% of common seaweeds and coral causes bleaching and death to the coral via transfer of lipid-soluble metabolites.[104] Seaweed and algae proliferate given adequate nutrients and limited grazing by herbivores such as parrotfish.
Water temperature changes of more than 1–2 °C (1.8–3.6 °F) or salinity changes can kill some species of coral. Under such environmental stresses, corals expel their Symbiodinium; without them, coral tissues reveal the white of their skeletons, an event known as coral bleaching.[105]
Submarine springs found along the coast of Mexico's Yucatán Peninsula produce water with a naturally low pH (relatively high acidity) providing conditions similar to those expected to become widespread as the oceans absorb carbon dioxide.[106] Surveys discovered multiple species of live coral that appeared to tolerate the acidity. The colonies were small and patchily distributed and had not formed structurally complex reefs such as those that compose the nearby Mesoamerican Barrier Reef System.[106]
Coral health
[edit]To assess the threat level of coral, scientists developed a coral imbalance ratio, Log (Average abundance of disease-associated taxa / Average abundance of healthy associated taxa). The lower the ratio the healthier the microbial community is. This ratio was developed after the microbial mucus of coral was collected and studied.[107]
Climate change impacts
[edit]Increasing sea surface temperatures in tropical regions (~1 °C (1.8 °F)) the last century have caused major coral bleaching, death, and therefore shrinking coral populations. Although coral are able to adapt and acclimate, it is uncertain if this evolutionary process will happen quickly enough to prevent major reduction of their numbers.[108] Climate change causes more frequent and more severe storms that can destroy coral reefs.[109]
Annual growth bands in some corals, such as the deep sea bamboo corals (Isididae), may be among the first signs of the effects of ocean acidification on marine life.[110] The growth rings allow geologists to construct year-by-year chronologies, a form of incremental dating, which underlie high-resolution records of past climatic and environmental changes using geochemical techniques.[111]
Certain species form communities called microatolls, which are colonies whose top is dead and mostly above the water line, but whose perimeter is mostly submerged and alive. Average tide level limits their height. By analyzing the various growth morphologies, microatolls offer a low-resolution record of sea level change. Fossilized microatolls can also be dated using radiocarbon dating. Such methods can help to reconstruct Holocene sea levels.[112]
Though coral have large sexually-reproducing populations, their evolution can be slowed by abundant asexual reproduction.[113] Gene flow is variable among coral species.[113] According to the biogeography of coral species, gene flow cannot be counted on as a dependable source of adaptation as they are very stationary organisms. Also, coral longevity might factor into their adaptivity.[113]
However, adaptation to climate change has been demonstrated in many cases, which is usually due to a shift in coral and zooxanthellae genotypes. These shifts in allele frequency have progressed toward more tolerant types of zooxanthellae.[114] Scientists found that a certain scleractinian zooxanthella is becoming more common where sea temperature is high.[115][116] Symbionts able to tolerate warmer water seem to photosynthesise more slowly, implying an evolutionary trade-off.[116]
In the Gulf of Mexico, where sea temperatures are rising, cold-sensitive staghorn and elkhorn coral have shifted in location.[114] Not only have the symbionts and specific species been shown to shift, but there seems to be a certain growth rate favorable to selection. Slower-growing but more heat-tolerant corals have become more common.[117] The changes in temperature and acclimation are complex. Some reefs in current shadows represent a refugium location that will help them adjust to the disparity in the environment even if eventually the temperatures may rise more quickly there than in other locations.[118] This separation of populations by climatic barriers causes a realized niche to shrink greatly in comparison to the old fundamental niche.
Geochemistry
[edit]Corals are shallow, colonial organisms that integrate oxygen and trace elements into their skeletal aragonite (polymorph of calcite) crystalline structures as they grow. Geochemical anomalies within the crystalline structures of corals represent functions of temperature, salinity and oxygen isotopic composition. Such geochemical analysis can help with climate modeling.[119] The ratio of oxygen-18 to oxygen-16 (δ18O), for example, is a proxy for temperature.
Strontium/calcium ratio anomaly
[edit]Time can be attributed to coral geochemistry anomalies by correlating strontium/calcium minimums with sea surface temperature (SST) maximums to data collected from NINO 3.4 SSTA.[120]
Oxygen isotope anomaly
[edit]The comparison of coral strontium/calcium minimums with sea surface temperature maximums, data recorded from NINO 3.4 SSTA, time can be correlated to coral strontium/calcium and δ18O variations. To confirm the accuracy of the annual relationship between Sr/Ca and δ18O variations, a perceptible association to annual coral growth rings confirms the age conversion. Geochronology is established by the blending of Sr/Ca data, growth rings, and stable isotope data. El Nino-Southern Oscillation (ENSO) is directly related to climate fluctuations that influence coral δ18O ratio from local salinity variations associated with the position of the South Pacific convergence zone (SPCZ) and can be used for ENSO modeling.[120]
Sea surface temperature and sea surface salinity
[edit]
The global moisture budget is primarily being influenced by tropical sea surface temperatures from the position of the Intertropical Convergence Zone (ITCZ).[121] The Southern Hemisphere has a unique meteorological feature positioned in the southwestern Pacific Basin called the South Pacific Convergence Zone (SPCZ), which contains a perennial position within the Southern Hemisphere. During ENSO warm periods, the SPCZ reverses orientation extending from the equator down south through Solomon Islands, Vanuatu, Fiji and towards the French Polynesian Islands; and due east towards South America affecting geochemistry of corals in tropical regions.[122]
Geochemical analysis of skeletal coral can be linked to sea surface salinity (SSS) and sea surface temperature (SST), from El Nino 3.4 SSTA data, of tropical oceans to seawater δ18O ratio anomalies from corals. ENSO phenomenon can be related to variations in sea surface salinity (SSS) and sea surface temperature (SST) that can help model tropical climate activities.[123]
Limited climate research on current species
[edit]
Climate research on live coral species is limited to a few studied species. Studying Porites coral provides a stable foundation for geochemical interpretations that is much simpler to physically extract data in comparison to Platygyra species where the complexity of Platygyra species skeletal structure creates difficulty when physically sampled, which happens to be one of the only multidecadal living coral records used for coral paleoclimate modeling.[123]
Protection
[edit]Marine Protected Areas, Biosphere reserves, marine parks, national monuments world heritage status, fishery management and habitat protection can protect reefs from anthropogenic damage.[124]
Many governments now prohibit removal of coral from reefs, and inform coastal residents about reef protection and ecology. While local action such as habitat restoration and herbivore protection can reduce local damage, the longer-term threats of acidification, temperature change and sea-level rise remain a challenge.[96]
Protecting networks of diverse and healthy reefs, not only climate refugia, helps ensure the greatest chance of genetic diversity, which is critical for coral to adapt to new climates.[125] A variety of conservation methods applied across marine and terrestrial threatened ecosystems makes coral adaption more likely and effective.[125]
To eliminate destruction of corals in their indigenous regions, projects have been started to grow corals in non-tropical countries.[126][127]
Relation to humans
[edit]Local economies near major coral reefs benefit from an abundance of fish and other marine creatures as a food source. Reefs also provide recreational scuba diving and snorkeling tourism. These activities can damage coral but international projects such as Green Fins that encourage dive and snorkel centres to follow a Code of Conduct have been proven to mitigate these risks.[128]
Jewelry
[edit]
Corals' many colors give it appeal for necklaces and other jewelry. Intensely red coral is prized as a gemstone. Sometimes called fire coral, it is not the same as fire coral. Red coral is very rare because of overharvesting.[129] In general, it is inadvisable to give coral as gifts since they are in decline from stressors like climate change, pollution, and unsustainable fishing.
Always considered a precious mineral, "the Chinese have long associated red coral with auspiciousness and longevity because of its color and its resemblance to deer antlers (so by association, virtue, long life, and high rank".[130] It reached its height of popularity during the Manchu or Qing Dynasty (1644–1911) when it was almost exclusively reserved for the emperor's use either in the form of coral beads (often combined with pearls) for court jewelry or as decorative Penjing (decorative miniature mineral trees). Coral was known as shanhu in Chinese. The "early-modern 'coral network' [began in] the Mediterranean Sea [and found its way] to Qing China via the English East India Company".[131] There were strict rules regarding its use in a code established by the Qianlong Emperor in 1759.
Medicine
[edit]
In medicine, chemical compounds from corals can potentially be used to treat cancer, neurological diseases, inflammation including arthritis, pain, bone loss, high blood pressure and for other therapeutic uses.[133][134] Coral skeletons, e.g. Isididae are being researched for their potential near-future use for bone grafting in humans.[135] Coral Calx, known as Praval Bhasma in Sanskrit, is widely used in traditional system of Indian medicine as a supplement in the treatment of a variety of bone metabolic disorders associated with calcium deficiency.[136] In classical times ingestion of pulverized coral, which consists mainly of the weak base calcium carbonate, was recommended for calming stomach ulcers by Galen and Dioscorides.[137]
Construction
[edit]Coral reefs in places such as the East African coast are used as a source of building material.[138] Ancient (fossil) coral limestone, notably including the Coral Rag Formation of the hills around Oxford (England), was once used as a building stone, and can be seen in some of the oldest buildings in that city including the Saxon tower of St Michael at the Northgate, St. George's Tower of Oxford Castle, and the medieval walls of the city.[139]
Shoreline protection
[edit]Healthy coral reefs absorb 97 percent of a wave's energy, which buffers shorelines from currents, waves, and storms, helping to prevent loss of life and property damage. Coastlines protected by coral reefs are also more stable in terms of erosion than those without.[140]
Local economies
[edit]Coastal communities near coral reefs rely heavily on them. Worldwide, more than 500 million people depend on coral reefs for food, income, coastal protection, and more.[141] The total economic value of coral reef services in the United States – including fisheries, tourism, and coastal protection – is more than $3.4 billion a year.
Aquaria
[edit]
The saltwater fishkeeping hobby has expanded, over recent years, to include reef tanks, fish tanks that include large amounts of live rock on which coral is allowed to grow and spread.[142] These tanks are either kept in a natural-like state, with algae (sometimes in the form of an algae scrubber) and a deep sand bed providing filtration,[143] or as "show tanks", with the rock kept largely bare of the algae and microfauna that would normally populate it,[144] in order to appear neat and clean.
The most popular kind of coral kept is soft coral, especially zoanthids and mushroom corals, which are especially easy to grow and propagate in a wide variety of conditions, because they originate in enclosed parts of reefs where water conditions vary and lighting may be less reliable and direct.[145] More serious fishkeepers may keep small polyp stony coral, which is from open, brightly lit reef conditions and therefore much more demanding, while large polyp stony coral is a sort of compromise between the two.
Aquaculture
[edit]Coral aquaculture, also known as coral farming or coral gardening, is the cultivation of corals for commercial purposes or coral reef restoration. Aquaculture is showing promise as a potentially effective tool for restoring coral reefs, which have been declining around the world.[146][147][148] The process bypasses the early growth stages of corals when they are most at risk of dying. Coral fragments known as "seeds" are grown in nurseries then replanted on the reef.[149] Coral is farmed by coral farmers who live locally to the reefs and farm for reef conservation or for income. It is also farmed by scientists for research, by businesses for the supply of the live and ornamental coral trade and by private aquarium hobbyists.
Gallery
[edit]Further images: commons:Category:Coral reefs and commons:Category:Corals
-
Fungia sp. skeleton
-
Polyps of Eusmilia fastigiata
-
Pillar coral, Dendrogyra cylindricus
-
Brain coral releasing eggs
See also
[edit]References
[edit]- ^ Rouzé, Héloïse; Galand, Pierre E.; Medina, Mónica; Bongaerts, Pim; Pichon, Michel; Pérez-Rosales, Gonzalo; Torda, Gergely; Moya, Aurelie; Raina, Jean-Baptiste; Hédouin, Laetitia (May 9, 2021). "Symbiotic associations of the deepest recorded photosynthetic scleractinian coral (172 m depth)". The ISME Journal. 15 (5): 1564–1568. Bibcode:2021ISMEJ..15.1564R. doi:10.1038/s41396-020-00857-y. PMC 8115523. PMID 33452473.
- ^ Squires, D.F. (1959). "Deep sea corals collected by the Lamont Geological Observatory. 1. Atlantic corals" (PDF). American Museum Novitates (1965): 23.
- ^ Leroi, Armand Marie (2014). The Lagoon: How Aristotle Invented Science. Bloomsbury. p. 271. ISBN 978-1-4088-3622-4.
- ^ a b c Bowen, James (2015). The Coral Reef Era: From Discovery to Decline: A history of scientific investigation from 1600 to the Anthropocene Epoch. Springer. pp. 5–7. ISBN 978-3-319-07479-5.
- ^ Babylonian Talmud, Rosh Hashana 23a, and commentary of Rashi (24th narrow line)
- ^ Egerton, Frank N. (2012). Roots of Ecology: Antiquity to Hackel. University of California Press. p. 24. ISBN 978-0-520-95363-5.
- ^ Swett, C. (5 March 2020). Corals: Secrets of Their Reef-Making Colonies. Capstone Global Library Ltd. ISBN 9781474771009.
- ^ Hoeksema, Bert (2015). "Anthozoa". WoRMS. World Register of Marine Species. Retrieved 2015-04-24.
- ^ a b c d Ramírez-Portilla, Catalina; Baird, Andrew H; Cowman, Peter F; Quattrini, Andrea M; Harii, Saki; Sinniger, Frederic; Flot, Jean-François (2022-03-01). "Solving the Coral Species Delimitation Conundrum". Systematic Biology. 71 (2): 461–475. doi:10.1093/sysbio/syab077. ISSN 1063-5157. PMID 34542634.
- ^ Ritchie, Hannah; Roser, Max (2021-04-15). "Biodiversity". Our World in Data.
- ^ Hume, Benjamin C. C.; D'Angelo, Cecilia; Burt, John A.; Wiedenmann, Jörg (2018). "Fine-Scale Biogeographical Boundary Delineation and Sub-population Resolution in the Symbiodinium thermophilum Coral Symbiont Group From the Persian/Arabian Gulf and Gulf of Oman". Frontiers in Marine Science. 5. doi:10.3389/fmars.2018.00138. hdl:10754/627647. ISSN 2296-7745.
- ^ a b c d e f g h i j k l m Hemond, Elizabeth M; Kaluziak, Stefan T; Vollmer, Steven V (2014-12-17). "The genetics of colony form and function in Caribbean Acropora corals". BMC Genomics. 15: 1133. doi:10.1186/1471-2164-15-1133. ISSN 1471-2164. PMC 4320547. PMID 25519925.
- ^ a b US Department of Commerce, National Oceanic and Atmospheric Administration. "NOAA's Coral Reef Conservation Program (CRCP) – Coral Facts". coralreef.noaa.gov. Retrieved 2022-04-26.
- ^ a b "Soft Corals: They Look Like Plants But Are Actually Animals". ThoughtCo. Retrieved 2022-04-26.
- ^ DeVictor, Susan T.; Morton, Steve L. (2010). "Octocoral Morphology". Guide to the Shallow-Water (0-200m) Octocorals of the South Atlantic Bight. Auckland, NZ: Magnolia Press. ISBN 978-1-86977-584-1.
- ^ Gilbert, Scott F (2000). "Embryonic Development". Developmental Biology (6th ed.). Sunderland, MA: Sinauer Associates. ISBN 0-87893-243-7.
- ^ Eric M, Engstrom; Izhaki, Anat; Bowman, John L (July 2004). "Promoter Bashing, microRNAs, and Knox Genes. New Insights, Regulators, and Targets-of-Regulation in the Establishment of Lateral Organ Polarity in Arabidopsis". Plant Physiology. 135 (2): 685–694. doi:10.1104/pp.104.040394. PMC 514105. PMID 15208415 – via ResearchGate.
- ^ Stemmer, Kristina; Burghardt, Ingo; Mayer, Christoph; Reinicke, Götz B.; Wägele, Heike; Tollrian, Ralph; Leese, Florian (June 2013). "Morphological and genetic analyses of xeniid soft coral diversity (Octocorallia; Alcyonacea)". Organisms Diversity & Evolution. 13 (2): 135–150. Bibcode:2013ODivE..13..135S. doi:10.1007/s13127-012-0119-x. ISSN 1439-6092. S2CID 17511020.
- ^ "Coral Reefs". IUCN. Archived from the original on 2022-04-27. Retrieved 2022-04-27.
- ^ a b Strauss, Chloe; Long, Hongan; Patterson, Caitlyn E.; Te, Ronald; Lynch, Michael (2017-09-06). Moran, Nancy A. (ed.). "Genome-Wide Mutation Rate Response to pH Change in the Coral Reef Pathogen Vibrio shilonii AK1". mBio. 8 (4): e01021–17. doi:10.1128/mBio.01021-17. ISSN 2161-2129. PMC 5565966. PMID 28830944.
- ^ a b c Ruppert, Edward E.; Fox, Richard S.; Barnes, Robert D. (2004). Invertebrate Zoology, 7th edition. Cengage Learning. pp. 132–48. ISBN 978-81-315-0104-7.
- ^ a b c d public domain: Minchin, Edward Alfred (1911). "Polyp". In Chisholm, Hugh (ed.). Encyclopædia Britannica. Vol. 22 (11th ed.). Cambridge University Press. p. 37. One or more of the preceding sentences incorporates text from a publication now in the
- ^ D. Gateno; A. Israel; Y. Barki; B. Rinkevich (1998). "Gastrovascular Circulation in an Octocoral: Evidence of Significant Transport of Coral and Symbiont Cells". The Biological Bulletin. 194 (2): 178–86. doi:10.2307/1543048. JSTOR 1543048. PMID 28570841. S2CID 19530967.
- ^ Peel, John S. (2017). "A problematic cnidarian (Cambroctoconus; Octocorallia?) from the Cambrian (Series 2–3) of Laurentia". Journal of Paleontology. 91 (5): 871–882. Bibcode:2017JPal...91..871P. doi:10.1017/jpa.2017.49. S2CID 134826884.
- ^ Administration, US Department of Commerce, National Oceanic and Atmospheric. "existing and potential value of coral ecosystems with respect to income and other economic values". coralreef.noaa.gov. Archived from the original on 2018-02-05. Retrieved 2018-02-04.
{{cite web}}
: CS1 maint: multiple names: authors list (link) - ^ Sprung, Julian (1999). Corals: A quick reference guide. Ricordea Publishing. p. 145. ISBN 978-1-883693-09-1.
- ^ Cuif, J.P.; Dauphin, Y. (1998). "Microstructural and physico-chemical characterization of 'centers of calcification' in septa of some Recent scleractinian corals". Paläontologische Zeitschrift. 72 (3–4): 257–269. Bibcode:1998PalZ...72..257C. doi:10.1007/bf02988357. ISSN 0031-0220. S2CID 129021387.
- ^ Cuif, J.P.; Dauphin, Y.; Doucet, J.; Salomé, M.; Susini, J. (2003). "XANES mapping of organic sulfate in three scleractinian coral skeletons". Geochimica et Cosmochimica Acta. 67 (1): 75–83. Bibcode:2003GeCoA..67...75C. doi:10.1016/s0016-7037(02)01041-4. ISSN 0016-7037.
- ^ Dauphin, Y.; Cuif, J.P.; Williams, C. T. (2008). "Soluble organic matrices of aragonitic skeletons of Merulinidae (Cnidaria, Anthozoa)". Comparative Biochemistry and Physiology Part B: Biochemistry and Molecular Biology. 150 (1): 10–22. doi:10.1016/j.cbpb.2008.01.002. ISSN 1096-4959. PMID 18325807.
- ^ Cuif, J.P.; Dauphin, Y.; Freiwald, A.; Gautret, P.; Zibrowius, H. (1999). "Biochemical markers of zooxanthellae symbiosis in soluble matrices of skeleton of 24 Scleractinia species". Comparative Biochemistry and Physiology Part A: Molecular & Integrative Physiology. 123 (3): 269–278. doi:10.1016/s1095-6433(99)00059-8. ISSN 1095-6433.
- ^ a b Murphy, Richard C. (2002). Coral Reefs: Cities Under The Seas. The Darwin Press. ISBN 978-0-87850-138-0.
- ^ Yuyama, Ikuko (2014). "Comparing the Effects of Symbiotic Algae (Symbiodinium) Clades C1 and D on Early Growth Stages of Acropora tenuis". PLOS ONE. 9 (6): e98999. Bibcode:2014PLoSO...998999Y. doi:10.1371/journal.pone.0098999. PMC 4051649. PMID 24914677.
- ^ Yamashita, Hiroshi (2014). "Establishment of Coral–Algal Symbiosis Requires Attraction and Selection". PLOS ONE. 9 (5): e97003. Bibcode:2014PLoSO...997003Y. doi:10.1371/journal.pone.0097003. PMC 4019531. PMID 24824794.
- ^ "Zooxanthellae...What's That?". NOAA Ocean Service Education. National Oceanic and Atmospheric Administration. Archived from the original on 13 April 2020. Retrieved 1 December 2017.
- ^ a b Madl, P.; Yip, M. (2000). "Field Excursion to Milne Bay Province – Papua New Guinea". Archived from the original on 2020-05-11. Retrieved 2006-03-31.
- ^ van de Plaasche, Orson (1986). Sea-level research: a manual for the collection and evaluation of data. Norwich, UK: Geo Books. p. 196. ISBN 978-94-010-8370-6.
- ^ "Corals and their microbiomes evolved together | Penn State University". psu.edu.
- ^ W. W. Toller; R. Rowan; N. Knowlton (2001). "Repopulation of Zooxanthellae in the Caribbean Corals Montastraea annularis and M. faveolata following Experimental and Disease-Associated Bleaching". The Biological Bulletin. 201 (3): 360–73. doi:10.2307/1543614. JSTOR 1543614. PMID 11751248. S2CID 7765487. Archived from the original on 2006-02-25. Retrieved 2006-03-30.
- ^ Brownlee, Colin (2009). "pH regulation in symbiotic anemones and corals: A delicate balancing act". Proceedings of the National Academy of Sciences of the United States of America. 106 (39): 16541–16542. Bibcode:2009PNAS..10616541B. doi:10.1073/pnas.0909140106. PMC 2757837. PMID 19805333.
- ^ Landsberg et al., "Stony Coral Tissue Loss Disease in Florida Is Associated With Disruption of Host–Zooxanthellae Physiology".
- ^ de O Santos et al., "Genomic and Proteomic Analyses of the Coral Pathogen Vibrio Coralliilyticus Reveal a Diverse Virulence Repertoire".
- ^ Avise, John C. (2011-03-18). Hermaphroditism: A Primer on the Biology, Ecology, and Evolution of Dual Sexuality. Columbia University Press. p. 83. ISBN 978-0-231-52715-6.
- ^ a b c d e f Markandeya, Virat (22 February 2023). "How lunar cycles guide the spawning of corals, worms and more". Knowable Magazine. Annual Reviews. doi:10.1146/knowable-022223-2. S2CID 257126558. Retrieved 6 March 2023.
- ^ a b c d Häfker, N. Sören; Andreatta, Gabriele; Manzotti, Alessandro; Falciatore, Angela; Raible, Florian; Tessmar-Raible, Kristin (16 January 2023). "Rhythms and Clocks in Marine Organisms". Annual Review of Marine Science. 15 (1): 509–538. Bibcode:2023ARMS...15..509H. doi:10.1146/annurev-marine-030422-113038. ISSN 1941-1405. PMID 36028229. S2CID 251865474.
- ^ a b c Veron, J.E.N. (2000). Corals of the World. Vol 3 (3rd ed.). Australia: Australian Institute of Marine Sciences and CRR Qld. ISBN 978-0-642-32236-4.
- ^ Hatta, M.; Fukami, H.; Wang, W.; Omori, M.; Shimoike, K.; Hayashibara, T.; Ina, Y.; Sugiyama, T. (1999). "Reproductive and genetic evidence for a reticulate evolutionary theory of mass spawning corals". Molecular Biology and Evolution. 16 (11): 1607–13. doi:10.1093/oxfordjournals.molbev.a026073. PMID 10555292.
- ^ a b Lin, Che-Hung; Takahashi, Shunichi; Mulla, Aziz J.; Nozawa, Yoko (24 August 2021). "Moonrise timing is key for synchronized spawning in coral Dipsastraea speciosa". Proceedings of the National Academy of Sciences. 118 (34): e2101985118. Bibcode:2021PNAS..11801985L. doi:10.1073/pnas.2101985118. ISSN 0027-8424. PMC 8403928. PMID 34373318.
- ^ Jabr, Ferris (June 13, 2017). "The Lunar Sea". Hakai Magazine. Retrieved 6 March 2023.
- ^ a b Barnes, R. and; Hughes, R. (1999). An Introduction to Marine Ecology (3rd ed.). Malden, MA: Blackwell. pp. 117–41. ISBN 978-0-86542-834-8.
- ^ Cameron, Kerry A.; Harrison, Peter L. (26 March 2020). "Density of coral larvae can influence settlement, post-settlement colony abundance and coral cover in larval restoration". Scientific Reports. 10 (1): 5488. Bibcode:2020NatSR..10.5488C. doi:10.1038/s41598-020-62366-4. ISSN 2045-2322. PMC 7099096. PMID 32218470.
- ^ Ayalon, Inbal; Rosenberg, Yaeli; Benichou, Jennifer I. C.; Campos, Celine Luisa D.; Sayco, Sherry Lyn G.; Nada, Michael Angelou L.; Baquiran, Jake Ivan P.; Ligson, Charlon A.; Avisar, Dror; Conaco, Cecilia; Kuechly, Helga U.; Kyba, Christopher C. M.; Cabaitan, Patrick C.; Levy, Oren (25 January 2021). "Coral Gametogenesis Collapse under Artificial Light Pollution". Current Biology. 31 (2): 413–419.e3. Bibcode:2021CBio...31E.413A. doi:10.1016/j.cub.2020.10.039. ISSN 0960-9822. PMID 33157030. S2CID 226257589.
- ^ Suzuki, Go; Okada, Wataru; Yasutake, Yoko; Yamamoto, Hidekazu; Tanita, Iwao; Yamashita, Hiroshi; Hayashibara, Takeshi; Komatsu, Toshiaki; Kanyama, Toru; Inoue, Masahito; Yamazaki, Masashi (September 2020). "Enhancing coral larval supply and seedling production using a special bundle collection system "coral larval cradle" for large-scale coral restoration". Restoration Ecology. 28 (5): 1172–1182. Bibcode:2020ResEc..28.1172S. doi:10.1111/rec.13178. ISSN 1061-2971. S2CID 218796945.
- ^ Jones, O.A.; Endean, R. (1973). Biology and Geology of Coral Reefs. New York, US: Harcourt Brace Jovanovich. pp. 205–45. ISBN 978-0-12-389602-5.
- ^ HARRISON, P. L; WALLACE, C. C. (1990). "Reproduction, dispersal and recruitment of scleractinian corals". Reproduction, Dispersal and Recruitment of Scleractinian Corals. 25: 133–207. ISSN 0167-4579.
- ^ Morse, Daniel E.; Hooker, Neal; Morse, Aileen N. C.; Jensen, Rebecca A. (1988-05-24). "Control of larval metamorphosis and recruitment in sympatric agariciid corals". Journal of Experimental Marine Biology and Ecology. 116 (3): 193–217. Bibcode:1988JEMBE.116..193M. doi:10.1016/0022-0981(88)90027-5. ISSN 0022-0981.
- ^ Webster, Nicole S.; Smith, Luke D.; Heyward, Andrew J.; Watts, Joy E. M.; Webb, Richard I.; Blackall, Linda L.; Negri, Andrew P. (2004-02-01). "Metamorphosis of a Scleractinian Coral in Response to Microbial Biofilms". Applied and Environmental Microbiology. 70 (2): 1213–1221. Bibcode:2004ApEnM..70.1213W. doi:10.1128/AEM.70.2.1213-1221.2004. ISSN 0099-2240. PMC 348907. PMID 14766608.
- ^ Ricardo, Gerard F.; Jones, Ross J.; Nordborg, Mikaela; Negri, Andrew P. (2017-12-31). "Settlement patterns of the coral Acropora millepora on sediment-laden surfaces". Science of the Total Environment. 609: 277–288. Bibcode:2017ScTEn.609..277R. doi:10.1016/j.scitotenv.2017.07.153. ISSN 0048-9697. PMID 28750231.
- ^ Birrell, CL; McCook, LJ; Willis, BL; Harrington, L (2008-06-30). "Chemical effects of macroalgae on larval settlement of the broadcast spawning coral Acropora millepora". Marine Ecology Progress Series. 362: 129–137. Bibcode:2008MEPS..362..129B. doi:10.3354/meps07524. ISSN 0171-8630.
- ^ Gulko, David (1998). Hawaiian Coral Reef Ecology. Honolulu, Hawaii: Mutual Publishing. p. 10. ISBN 978-1-56647-221-0.
- ^ Sheppard, Charles R.C.; Davy, Simon K.; Pilling, Graham M. (25 June 2009). The Biology of Coral Reefs. OUP Oxford. pp. 78–81. ISBN 978-0-19-105734-2.
- ^ Sharp, Koty H.; Pratte, Zoe A.; Kerwin, Allison H.; Rotjan, Randi D.; Stewart, Frank J. (2017). "Season, but not symbiont state, drives microbiome structure in the temperate coral Astrangia poculata". Microbiome. 5 (1): 120. doi:10.1186/s40168-017-0329-8. PMC 5603060. PMID 28915923.
- ^ Dubinsky, Z. and Jokiel, P.L. (1994) "Ratio of energy and nutrient fluxes regulates symbiosis between zooxanthellae and corals". Pacific Science, 48(3): 313–324.
- ^ Anthony, K.R., Kline, D.I., Diaz-Pulido, G., Dove, S. and Hoegh-Guldberg, O.(2008) "Ocean acidification causes bleaching and productivity loss in coral reef builders". Proceedings of the National Academy of Sciences, 105(45): 17442–17446. doi:10.1073/pnas.0804478105.
- ^ a b Apprill, A. (2017) "Marine animal microbiomes: toward understanding host–microbiome interactions in a changing ocean". Frontiers in Marine Science, 4: 222. doi:10.3389/fmars.2017.00222.
Material was copied from this source, which is available under a Creative Commons Attribution 4.0 International License.
- ^ Bourne, D.G., Morrow, K.M. and Webster, N.S. (2016) "Insights into the coral microbiome: underpinning the health and resilience of reef ecosystems". Annual Review of Microbiology, 70: 317–340. doi:10.1146/annurev-micro-102215-095440.
- ^ Neave, M.J., Apprill, A., Ferrier-Pagès, C. and Voolstra, C.R. (2016) "Diversity and function of prevalent symbiotic marine bacteria in the genus Endozoicomonas". Applied Microbiology and Biotechnology, 100(19): 8315–8324. doi:10.1007/s00253-016-7777-0.
- ^ Neave, M.J., Michell, C.T., Apprill, A. and Voolstra, C.R. (2017) "Endozoicomonas genomes reveal functional adaptation and plasticity in bacterial strains symbiotically associated with diverse marine hosts". Scientific Reports, 7: 40579. doi:10.1038/srep40579.
- ^ Hughes, T.P., Kerry, J.T., Álvarez-Noriega, M., Álvarez-Romero, J.G., Anderson, K.D., Baird, A.H., Babcock, R.C., Beger, M., Bellwood, D.R., Berkelmans, R. and Bridge, T.C. (2017) "Global warming and recurrent mass bleaching of corals". Nature, 543(7645): 373–377. doi:10.1038/nature21707.
- ^ USGS scientists publish long-read microbiome sequences from temperate coral, providing community resource for probe and primer design, United States Geological Survey, 6 March 2019.
Material was copied from this source, which is available under a Creative Commons Attribution 4.0 International License.
- ^ b. Goldsmith, Dawn; a. Pratte, Zoe; a. Kellogg, Christina; e. Snader, Sara; h. Sharp, Koty (2019). "Stability of temperate coral Astrangia poculata microbiome is reflected across different sequencing methodologies". AIMS Microbiology. 5 (1): 62–76. doi:10.3934/microbiol.2019.1.62. PMC 6646935. PMID 31384703.
Material was copied from this source, which is available under a Creative Commons Attribution 4.0 International License.
- ^ Knowlton, N. and Rohwer, F. (2003) "Multispecies microbial mutualisms on coral reefs: the host as a habitat". The American Naturalist, 162(S4): S51-S62. doi:10.1086/378684.
- ^ Pollock, F. Joseph; McMinds, Ryan; Smith, Styles; Bourne, David G.; Willis, Bette L.; Medina, Mónica; Thurber, Rebecca Vega; Zaneveld, Jesse R. (2018-11-22). "Coral-associated bacteria demonstrate phylosymbiosis and cophylogeny". Nature Communications. 9 (1): 4921. Bibcode:2018NatCo...9.4921P. doi:10.1038/s41467-018-07275-x. ISSN 2041-1723. PMC 6250698. PMID 30467310.
- ^ Peixoto, R.S., Rosado, P.M., Leite, D.C.D.A., Rosado, A.S. and Bourne, D.G. (2017) "Beneficial microorganisms for corals (BMC): proposed mechanisms for coral health and resilience". Frontiers in Microbiology, 8: 341. doi:10.3389/fmicb.2017.00341.
- ^ Apprill, Amy; Weber, Laura G.; Santoro, Alyson E. (2016). "Distinguishing between Microbial Habitats Unravels Ecological Complexity in Coral Microbiomes". mSystems. 1 (5): e00143–16. doi:10.1128/mSystems.00143-16. PMC 5080407. PMID 27822559.
- ^ La Rivière, Marie; Garrabou, Joaquim; Bally, Marc (2015-12-01). "Evidence for host specificity among dominant bacterial symbionts in temperate gorgonian corals". Coral Reefs. 34 (4): 1087–1098. Bibcode:2015CorRe..34.1087L. doi:10.1007/s00338-015-1334-7. ISSN 1432-0975. S2CID 14309443.
- ^ van de Water, Jeroen A. J. M.; Melkonian, Rémy; Voolstra, Christian R.; Junca, Howard; Beraud, Eric; Allemand, Denis; Ferrier-Pagès, Christine (2017-02-01). "Comparative Assessment of Mediterranean Gorgonian-Associated Microbial Communities Reveals Conserved Core and Locally Variant Bacteria". Microbial Ecology. 73 (2): 466–478. Bibcode:2017MicEc..73..466V. doi:10.1007/s00248-016-0858-x. ISSN 1432-184X. PMID 27726033. S2CID 22336906.
- ^ Pollock, F. Joseph; McMinds, Ryan; Smith, Styles; Bourne, David G.; Willis, Bette L.; Medina, Mónica; Thurber, Rebecca Vega; Zaneveld, Jesse R. (2018-11-22). "Coral-associated bacteria demonstrate phylosymbiosis and cophylogeny". Nature Communications. 9 (1): 4921. Bibcode:2018NatCo...9.4921P. doi:10.1038/s41467-018-07275-x. ISSN 2041-1723. PMC 6250698. PMID 30467310.
- ^ Thompson, J.R., Rivera, H.E., Closek, C.J. and Medina, M. (2015) "Microbes in the coral holobiont: partners through evolution, development, and ecological interactions". Frontiers in cellular and infection microbiology, 4: 176. doi:10.3389/fcimb.2014.00176.
Material was copied from this source, which is available under a Creative Commons Attribution 4.0 International License.
- ^ Thompson, J.R., Rivera, H.E., Closek, C.J. and Medina, M. (2015) "Microbes in the coral holobiont: partners through evolution, development, and ecological interactions". Frontiers in cellular and infection microbiology, 4: 176. doi:10.3389/fcimb.2014.00176.
- ^ Schuhmacher, Helmut; Zibrowius, Helmut (1985). "What is hermatypic?". Coral Reefs. 4 (1): 1–9. Bibcode:1985CorRe...4....1S. doi:10.1007/BF00302198. S2CID 34909110.
- ^ Great Barrier Reef. MSN Encarta. 2006. Archived from the original on October 28, 2009. Retrieved April 25, 2015.
- ^ Spalding, Mark; Ravilious, Corinna; Green, Edmund (2001). World Atlas of Coral Reefs. Berkeley, CA: University of California Press and UNEP/WCMC. pp. 205–45. ISBN 978-0-520-23255-6.
- ^ Smithsonian National Museum flickr.
- ^ Alden, Andrew. "Index Fossils". About education. Archived from the original on 1 December 2016. Retrieved 25 April 2015.
- ^ Pratt, B.R.; Spincer, B.R.; Wood, R.A.; Zhuravlev, A.Yu. (2001). "12: Ecology and Evolution of Cambrian Reefs" (PDF). Ecology of the Cambrian Radiation. Columbia University Press. p. 259. ISBN 978-0-231-10613-9. Retrieved 2007-04-06.[permanent dead link ]
- ^ Vinn, O.; Mõtus, M.-A. (2008). "The earliest endosymbiotic mineralized tubeworms from the Silurian of Podolia, Ukraine". Journal of Paleontology. 82 (2): 409–14. Bibcode:2008JPal...82..409V. doi:10.1666/07-056.1. S2CID 131651974. Retrieved 2014-06-11.
- ^ Vinn, O.; Mõtus, M.-A. (2012). "Diverse early endobiotic coral symbiont assemblage from the Katian (Late Ordovician) of Baltica". Palaeogeography, Palaeoclimatology, Palaeoecology. 321–322: 137–41. Bibcode:2012PPP...321..137V. doi:10.1016/j.palaeo.2012.01.028.
- ^ a b "Introduction to the Tabulata". UCMP Berkeley. Archived from the original on 19 April 2015. Retrieved 25 April 2015.
- ^ "Introduction to the Rugosa". UCMP Berkeley. Archived from the original on 19 April 2015. Retrieved 25 April 2015.
- ^ Xiang-Dong Wang and Xiao-Juan Wang (2007). "Extinction patterns of Late Permian (Lopingian) corals in China", Palaeoworld, 16, No. 1–3, 31–38
- ^ Ries JB, Stanley SM, Hardie LA (July 2006). "Scleractinian corals produce calcite, and grow more slowly, in artificial Cretaceous seawater". Geology. 34 (7): 525–28. Bibcode:2006Geo....34..525R. doi:10.1130/G22600.1.
- ^ "Evolutionary history". AIMS. Archived from the original on 13 October 2018. Retrieved 25 April 2015.
- ^ Waggoner, Ben M. (2000). Smith, David; Collins, Allen (eds.). "Anthozoa: Fossil Record". Anthozoa. UCMP. Retrieved 9 March 2020.
- ^ Oliver, William A. Jr. (2003). "Corals: Table 1". Fossil Groups. USGS. Archived from the original on 9 January 2009. Retrieved 9 March 2020.
- ^ "Coral reefs around the world". The Guardian. 2 September 2009.
- ^ a b "Threats to Coral Reefs". Coral Reef Alliance. 2010. Archived from the original on 1 December 2011. Retrieved 5 December 2011.
- ^ Losing Our Coral Reefs – Eco Matters – State of the Planet. Blogs.ei.columbia.edu. Retrieved on 2011-11-01.
- ^ Kleypas, J.A.; Feely, R.A.; Fabry, V.J.; Langdon, C.; Sabine, C.L.; Robbins, L.L. (2006). "Impacts of Ocean Acidification on Coral Reefs and Other Marine Calcifiers: A guide for Future Research" (PDF). National Science Foundation, NOAA, and United States Geological Survey. Archived from the original (PDF) on July 20, 2011. Retrieved April 7, 2011.
- ^ Save Our Seas, 1997 Summer Newsletter, Cindy Hunter and Alan Friedlander
- ^ Tun, K.; Chou, L.M.; Cabanban, A.; Tuan, V.S.; Philreefs, S.; Yeemin, T.; Suharsono; Sour, K.; Lane, D. (2004). "Status of Coral Reefs, Coral Reef Monitoring and Management in Southeast Asia, 2004". In Wilkinson, C. (ed.). Status of Coral Reefs of the world: 2004. Townsville, Queensland, Australia: Australian Institute of Marine Science. pp. 235–76. Retrieved 2019-04-23.
- ^ Burke, Lauretta; Reytar, K.; Spalding, M.; Perry, A. (2011). Reefs at risk revisited. Washington, DC: World Resources Institute. p. 38. ISBN 978-1-56973-762-0.
- ^ Bryant, Dirk; Burke, Lauretta; McManus, John; Spalding, Mark. "Reefs at Risk: A Map-Based Indicator of Threats to the World's Coral Reef" (PDF). NOAA. Archived from the original (PDF) on 2013-02-18. Retrieved 25 April 2015.
- ^ Norlander (8 December 2003). "Coral crisis! Humans are killing off these bustling underwater cities. Can coral reefs be saved? (Life science: corals)". Science World.
- ^ Rasher DB, Hay ME (May 2010). "Chemically rich seaweeds poison corals when not controlled by herbivores". Proceedings of the National Academy of Sciences of the United States of America. 107 (21): 9683–88. Bibcode:2010PNAS..107.9683R. doi:10.1073/pnas.0912095107. PMC 2906836. PMID 20457927.
- ^ Hoegh-Guldberg, O. (1999). "Climate change, coral bleaching and the future of the world's coral reefs". Marine and Freshwater Research. 50 (8): 839–66. doi:10.1071/MF99078.
- ^ a b Stephens, Tim (28 November 2011). "Submarine springs offer preview of ocean acidification effects on coral reefs". University of California Santa Cruz. Retrieved 25 April 2015.
- ^ "Health and Disease Signatures of the Coral Microbiome • iBiology". iBiology. Archived from the original on 2020-07-28. Retrieved 2020-05-14.
- ^ Hoegh-Guldberg O. (1999). "Climate change, coral bleaching and the future of the world's coral reefs". Marine and Freshwater Research. 50 (8): 839–99. doi:10.1071/mf99078 (inactive 16 December 2024).
{{cite journal}}
: CS1 maint: DOI inactive as of December 2024 (link) - ^ US Department of Commerce, National Oceanic and Atmospheric Administration. "How does climate change affect coral reefs?". oceanservice.noaa.gov.
- ^ "National Oceanic and Atmospheric Administration – New Deep-Sea Coral Discovered on NOAA-Supported Mission". noaanews.noaa.gov. Retrieved 2009-05-11.
- ^ Schrag, D.P.; Linsley, B.K. (2002). "Corals, chemistry, and climate". Science. 296 (8): 277–78. doi:10.1126/science.1071561. PMID 11951026. S2CID 82449130.
- ^ Smithers, Scott G.; Woodroffe, Colin D. (2000). "Microatolls as sea-level indicators on a mid-ocean atoll". Marine Geology. 168 (1–4): 61–78. Bibcode:2000MGeol.168...61S. doi:10.1016/S0025-3227(00)00043-8.
- ^ a b c Hughes, T.; Baird, A.; Bellwood, D.; Card, M.; Connolly, S.; Folke, C.; Grosberg, R.; Hoegh-Guldberg, O.; Jackson, J.; Klepas, J.; Lough, J.; Marshall, P.; Nystrom, M.; Palumbi, S.; Pandolfi, J.; Rosen, B.; and Roughgarden, J. (2003). "Climate change, human impacts, and the resilience of coral reefs". Science. 301 (5635): 929–33. Bibcode:2003Sci...301..929H. doi:10.1126/science.1085046. PMID 12920289. S2CID 1521635.
- ^ a b Parmesan, C. (2006). "Ecological and evolutionary responses to recent climate change". Annual Review of Ecology, Evolution, and Systematics. 37: 637–69. doi:10.1146/annurev.ecolsys.37.091305.110100.
- ^ Baker, A. (2004). "Corals' adaptive response to climate change". Nature. 430 (7001): 741. Bibcode:2004Natur.430..741B. doi:10.1038/430741a. PMID 15306799. S2CID 32092741.
- ^ a b Donner, S.; Skirving, W.; Little, C.; Oppenheimer, M.; Hoegh-Guldberg, O. (2005). "Global assessment of coral bleaching and required rates of adaptation under climate change" (PDF). Global Change Biology. 11 (12): 2251–65. Bibcode:2005GCBio..11.2251D. CiteSeerX 10.1.1.323.8134. doi:10.1111/j.1365-2486.2005.01073.x. PMID 34991281. S2CID 84890014. Archived from the original (PDF) on 2017-08-14. Retrieved 2017-10-25.
- ^ Baskett, M.; Gaines, S. & Nisbet, R. (2009). "Symbiont diversity may help coral reefs survive moderate climate change" (PDF). Ecological Applications. 19 (1): 3–17. Bibcode:2009EcoAp..19....3B. doi:10.1890/08-0139.1. PMID 19323170. S2CID 1189125.
- ^ McClanahan, T.; Ateweberhan, M.; Muhando, C.; Maina, J. & Mohammed, M. (2007). "Effects of Climate and Seawater Temperature Variation on Coral Bleaching and Morality". Ecological Monographs. 77 (4): 503–25. Bibcode:2007EcoM...77..503M. CiteSeerX 10.1.1.538.970. doi:10.1890/06-1182.1.
- ^ Kilbourne, K. Halimeda; Quinn, Terrence M.; Taylor, Frederick W.; Delcroix, Thierry; Gouriou, Yves (2004). "El Niño-Southern Oscillation-related salinity variations recorded in the skeletal geochemistry of a Porites coral from Espiritu Santo, Vanuatu". Paleoceanography. 19 (4): PA4002. Bibcode:2004PalOc..19.4002K. doi:10.1029/2004PA001033.
- ^ a b Ren, Lei; Linsley, Braddock K.; Wellington, Gerard M.; Schrag, Daniel P.; Hoegh-guldberg, Ove (2003). "Deconvolving the δ18O seawater component from subseasonal coral δ18O and Sr/Ca at Rarotonga in the southwestern subtropical Pacific for the period 1726 to 1997". Geochimica et Cosmochimica Acta. 67 (9): 1609–21. Bibcode:2003GeCoA..67.1609R. doi:10.1016/S0016-7037(02)00917-1.
- ^ Wu, Henry C.; Linsley, Braddock K.; Dassié, Emilie P.; Schiraldi, Benedetto; deMenocal, Peter B. (2013). "Oceanographic variability in the South Pacific Convergence Zone region over the last 210 years from multi-site coral Sr/Ca records". Geochemistry, Geophysics, Geosystems. 14 (5): 1435–53. Bibcode:2013GGG....14.1435W. doi:10.1029/2012GC004293.
- ^ Kiladis, George N.; von Storch, Hans; van Loon, Harry (1989). "Origin of the South Pacific Convergence Zone". Journal of Climate. 2 (10): 1185–95. Bibcode:1989JCli....2.1185K. doi:10.1175/1520-0442(1989)002<1185:OOTSPC>2.0.CO;2.
- ^ a b Lukas, Roger; Lindstrom, Eric (1991). "The mixed layer of the western equatorial Pacific Ocean". Journal of Geophysical Research. 96 (S1): 3343–58. Bibcode:1991JGR....96.3343L. doi:10.1029/90JC01951.
- ^ "Phoenix Rising". National Geographic Magazine. January 2011. Archived from the original on December 18, 2010. Retrieved April 30, 2011.
- ^ a b Walsworth, T.E.; Schindler, D.E.; Colton, M.A.; Webster, M.S.; Palumbi, S.R.; Mumby, P.J.; Essington, T.E.; Pinsky, M.L. (July 1, 2019). "Management for network diversity speeds evolutionary adaptation to climate change". Nature Research. 9: 632–636.
{{cite journal}}
: CS1 maint: multiple names: authors list (link) - ^ EcoDeco EcologicalTechnology Archived 2011-03-07 at the Wayback Machine. Ecodeco.nl. Retrieved on 2011-11-29.
- ^ KoralenKAS project Archived 2012-04-26 at the Wayback Machine. Koraalwetenschap.nl. Retrieved on 2011-11-29.
- ^ Hunt, Chloe V.; Harvey, James J.; Miller, Anne; Johnson, Vivienne; Phongsuwan, Niphon (2013). "The Green Fins approach for monitoring and promoting environmentally sustainable scuba diving operations in South East Asia". Ocean & Coastal Management. 78: 35–44. Bibcode:2013OCM....78...35H. doi:10.1016/j.ocecoaman.2013.03.004.
- ^ Magsaysay, Melissa (June 21, 2009). "Coral makes a splash". Los Angeles Times. Retrieved January 12, 2013.
- ^ Welch, Patricia Bjaaland, Chinese Art: A Guide to Motifs and Visual Imagery. Tokyo, Rutland and Singapore: Tuttle, 2008, p. 61
- ^ Lacey, Pippa, "The Coral Network: The trade of red coral to the Qing imperial court in the eighteenth century" in The Global Lives of Things, ed. by Anne Gerritsen and Giorgio Aiello, London: Rutledge, 2016, p. 81
- ^ Folio 391, Juliana Anicia Codex
- ^ Copper, Edwin; Hirabayashi, K.; Strychar, K. B.; Sammarco, P. W. (2014). "Corals and their Potential Applications to Integrative Medicine". Evidence-Based Complementary and Alternative Medicine. 2014: 9. doi:10.1155/2014/184959. PMC 3976867. PMID 24757491.
- ^ Senthilkumar, Kalimuthu; Se-Kwon, Kim (2013). "Marine Invertebrate Natural Products for Anti-Inflammatory and Chronic Diseases". Evidence-Based Complementary and Alternative Medicine. 2013: 572859. doi:10.1155/2013/572859. PMC 3893779. PMID 24489586.
- ^ Ehrlich, H.; Etnoyer, P.; Litvinov, S. D.; Olennikova, M.M.; Domaschke, H.; Hanke, T.; Born, R.; Meissner, H.; Worch, H. (2006). "Biomaterial structure in deep-sea bamboo coral (Anthozoa: Gorgonacea: Isididae): perspectives for the development of bone implants and templates for tissue engineering". Materialwissenschaft und Werkstofftechnik. 37 (6): 552–57. doi:10.1002/mawe.200600036. S2CID 97972721.
- ^ Reddy PN, Lakshmana M, Udupa UV (December 2003). "Effect of Praval bhasma (Coral calx), a natural source of rich calcium on bone mineralization in rats". Pharmacological Research. 48 (6): 593–99. doi:10.1016/S1043-6618(03)00224-X. PMID 14527824.
- ^ Pedanius Dioscorides – Der Wiener Dioskurides, Codex medicus Graecus 1 der Österreichischen Nationalbibliothek Graz: Akademische Druck- und Verlagsanstalt 1998 fol. 391 verso (Band 2), Kommentar S. 47 und 52. ISBN 3-201-01725-6
- ^ Pouwels, Randall L. (6 June 2002). Horn and Crescent: Cultural Change and Traditional Islam on the East African Coast, 800–1900. Cambridge University Press. p. 26. ISBN 978-0-521-52309-7.
- ^ "Strategic Stone Study: A Building Stone Atlas of Oxfordshire". English Heritage. March 2011. Retrieved 23 April 2015.
- ^ Ferrario, F.; Beck, M.W.; Storlazzi, C.D.; Micheli, F.; Shepard, C.C.; Airoldi, L. (2014). "The effectiveness of coral reefs for coastal hazard risk reduction and adaptation". Nature Communications. 5 (3794): 3794. Bibcode:2014NatCo...5.3794F. doi:10.1038/ncomms4794. PMC 4354160. PMID 24825660.
- ^ "Status of Coral Reefs of the World: 2004 Volume 1" (PDF). Global Coral Reef Monitoring Network. Archived from the original (PDF) on 2019-06-17. Retrieved 2019-01-14.
- ^ Aquarium Corals: Collection and Aquarium Husbandry of Northeast Pacific Non-Photosynthetic Cnidaria Archived 2017-06-06 at the Wayback Machine. Advancedaquarist.com (2011-01-14). Retrieved on 2016-06-13.
- ^ Reefkeeping 101 – Various Nutrient Control Methods. Reefkeeping.com. Retrieved on 2016-06-13.
- ^ Aquarium Substrate & Live Rock Clean Up Tips Archived 2016-08-06 at the Wayback Machine. Saltaquarium.about.com. Retrieved on 2016-06-13.
- ^ Coral Reefs Archived 2013-06-25 at archive.today. Marinebio.org. Retrieved on 2016-06-13.
- ^ Horoszowski-Fridman YB, Izhaki I, Rinkevich B (2011). "Engineering of coral reef larval supply through transplantation of nursery-farmed gravid colonies". Journal of Experimental Marine Biology and Ecology. 399 (2): 162–66. Bibcode:2011JEMBE.399..162H. doi:10.1016/j.jembe.2011.01.005.
- ^ Pomeroy, Robert S.; Parks, John E.; Balboa, Cristina M. (2006). "Farming the reef: Is aquaculture a solution for reducing fishing pressure on coral reefs?". Marine Policy. 30 (2): 111–30. Bibcode:2006MarPo..30..111P. doi:10.1016/j.marpol.2004.09.001.
- ^ Rinkevich B (2008). "Management of coral reefs: We have gone wrong when neglecting active reef restoration" (PDF). Marine Pollution Bulletin. 56 (11): 1821–24. Bibcode:2008MarPB..56.1821R. doi:10.1016/j.marpolbul.2008.08.014. PMID 18829052. Archived from the original (PDF) on 2013-05-23.
- ^ Ferse, Sebastian C.A. (2010). "Poor Performance of Corals Transplanted onto Substrates of Short Durability". Restoration Ecology. 18 (4): 399–407. Bibcode:2010ResEc..18..399F. doi:10.1111/j.1526-100X.2010.00682.x. S2CID 83723761.
Sources
[edit]- Allen, G.R.; R. Steene (1994). Indo-Pacific Coral Reef Field Guide. Tropical Reef Research. ISBN 978-981-00-5687-2.
- Calfo, Anthony (2007). Book of Coral Propagation. Reading Trees Publications. ISBN 978-0-9802365-0-7.
- Colin, P.L.; C. Arneson (1995). Tropical Pacific Invertebrates. Coral Reef Press. ISBN 978-0-9645625-0-9.
- Fagerstrom, J.A. (1987). The Evolution of Reef Communities. Wiley. ISBN 978-0-471-81528-0.
- Gosliner, T.; D. Behrens; G. Williams (1996). Coral Reef Animals of the Indo-Pacific, Animals Life from Africa to Hawai'i (invertebrates). Sea Challengers. ISBN 978-0-930118-21-1.
- Nybakken, J.W. (2004). Marine Biology, An Ecological Approach. Pearson/Benjamin Cummings. ISBN 978-0-8053-4582-7.
- Redhill, Surrey. Corals of the World: Biology and Field Guide.
- Segaloff, Nat; Paul Erickson (1991). A Reef Comes to Life. Creating an Undersea Exhibit. F. Watts. ISBN 978-0-531-10994-6.
- Sheppard, Charles R.C.; Davy, Simon K.; Pilling, Graham M. (25 June 2009). The Biology of Coral Reefs. OUP Oxford. ISBN 978-0-19-105734-2.
- Veron, J.E.N. (1993). Corals of Australia and the Indo-Pacific. University of Hawaii Press. ISBN 978-0-8248-1504-2.
- Wells, Susan (1988). Coral Reefs of the World. IUCN, UNEP. ISBN 9782880329440.
External links
[edit]- Coral Reefs The Ocean Portal by the Smithsonian Institution
- NOAA – Coral Reef Conservation Program
- NOAA CoRIS – Coral Reef Biology
- NOAA Office for Coastal Management – Fast Facts – Coral Reefs
- NOAA Ocean Service Education – Corals
- "What is a coral?". Stanford microdocs project. Archived from the original on 2014-01-06. Retrieved 2017-02-04.